Bitesize and Sounds revision podcasts | Overview
Revise GCSE Biology by listening to these podcasts from Bitesize and BBC Sounds.
BBC Sounds is where you can catch the latest music tracks, discover binge-worthy podcasts or listen to live radio, all in one place.Listen on the BBC Sounds app when you're out and about, or listen at home as part of your revision.
Let Dr Alex Lathbridge guide you through the key facts about cell structure
Supercharge your revision with more podcasts for GCSE English literature and GCSE Biology
Episodes are roughly ten minutes long and there are up to nine episodes in each series.
Making notes as you listen can help you remember the key points before the exam.
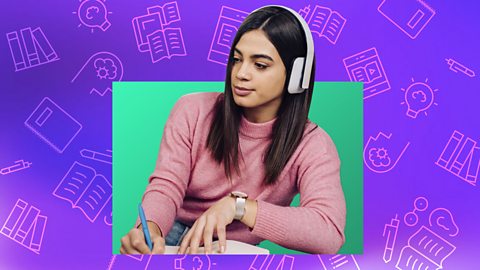
Episode 1 - Cell structure
Almost all animals and plants are made up of cells. This episode breaks down the key facts about cell structure.
Dr Alex Lathbridge explores the structure of cells.
Hello, I’m Dr Alex Lathbridge and this is Bitesize Biology.
In this podcast I’m going to take you through the main things you need to know for your GCSE Biology exam.
I’m going to be talking about things as small as cells, as big as rainforests and everything in-between.
There’s going to be a lot for you to remember. And as we go through all of the topics, there will be a few key terms that you’re going to need to learn off by heart. But don’t worry, I did it, you can do it too.
This is the is the first episode of a nine-part series on The Cell, let’s go.
All living things are made of cells, which is why they’re called the building blocks of life.
Plants are made of cells, animals are made of cells, humans are animals so even you, yes you, are made of cells.
In fact, right now, you are a giant, complex container made out of trillions of cells all working together, to learn about cells.
Anyway, today we’re going to take it back to basics and find out about the structure of the cell and the two main types that you are going to need to know.
The thing about cells is that they are small, like really, really small.
Anything between 0.01 and 0.1mm across.
This means they are too small to see with the naked eye. In order to study them properly, scientists first had to invent really powerful microscopes in order to see what’s inside them.
If this is all new to you, don’t worry, scientists like me are learning stuff all the time too.
Nearly all cells have four key things in common:
They have a membrane. This holds the cell together, keeps its contents inside and controls what can enter and exit the cell. Think of it like a balloon that can let things in and out.
They have a cytoplasm, which is kind of like a really useful jelly. It’s where all the chemical reactions take place in the cell. Think of it like water inside that balloon.
They have DNA. DNA is genetic material. And it contains all the information that tells the cell what to do, your cells following all these instructions is how you develop and grow.
They have ribosomes. These are sort of like mini-robots which make proteins based on the instructions found in DNA.
So, you could say that a simple cell is just a tiny a water balloon, filled with instructions and little protein-making robots.
Or, if you want to pass your exams, you could say that a cell is between 0.01 and 0.1mm across and made of a cell membrane containing cytoplasm, DNA and ribosomes.
Now, there are two types of cells you’ll need to know about:
Eukaryotic and prokaryotic cells.
You are a eukaryotic organism. This means that you are made up of lots of different types of cells, most of which have a nucleus.
These kinds of cells, the ones that work together with lots of other kinds of cells to make up a plant or animal or human, are called eukaryotic. Easy to remember: because you are eukaryotic.
Let’s think about most eukaryotic animal cells. As well as the stuff we’ve just talked about (that water balloon) most eukaryotic cells also have a nucleus.
Remember that cells always contain DNA? Well, in eukaryotic cells, the genetic material is kept inside a nucleus. Think of it like a little folder.
And they have Mitochondria. This is where cells release the energy they need, that’s where respiration takes place. Think of them like little batteries.
So, when it comes to most eukaryotic animal cells, we’ve got our water balloon (a membrane filled with cytoplasm), containing instructions to make proteins inside a little folder (DNA inside a nucleus), robots to synthesise the proteins (ribosomes) and batteries to power the whole thing (or mitochondria).
And just because things are never that simple, in animals red blood cells are the exception because they don’t have a nucleus.
It’s worth drawing it out, I've always found it useful, even just a quick doodle. Remember, there are lots of diagrams on the Bitesize website. It might be useful to look at those while you listen.
Now, although you might think you are a little bit more interesting than a lettuce, most eukaryotic cells in plants have some extra stuff on top of all that. So, plant cells have a membrane, cytoplasm, DNA, a nucleus and mitochondria and:
They have an extra layer outside of the cell membrane called the cell wall. Plants need a cell wall because they need to stand up straight, but they don’t have a skeleton, and this is where the cell wall comes in, it helps keep plants upright.
Green cells in plants’ stems and leaves have chloroplasts, which contain chlorophyll and the enzymes needed for photosynthesis. Photosynthesis is really important and we’re going to come back to it later in the series, but for now what you need to know is that chloroplasts contain the stuff that plant cells use to store energy from sunlight. Think of it like a little solar panel.
They also have a permanent vacuole. You can basically think of it like a little pocket inside of the cell, filled with something called cell sap that helps to keep the cell in shape.
And remember how red blood cells don’t have a nucleus? Most plant root cells don’t have chloroplasts, so they’re white not green.
Ok, so that was eukaryotic cells. Now let’s look at prokaryotic cells
Prokaryotic cells are always single celled organisms.
Like bacteria. The whole organism is just one tiny cell that does it all.
But just because it’s small doesn’t mean it’s not important. We need bacteria in our guts, to help us break down food and the environment is the same, the life cycle of things dying, and their nutrients being returned to the earth depends on bacteria.
This is why you should be very pro prokaryotic cells.
Like all cells, prokaryotes have a cell membrane, cytoplasm, DNA, and ribosomes.
However, there are two main differences:
Firstly, the DNA isn’t inside a nucleus, instead it floats free inside the cytoplasm in a single circular loop of DNA, with some extra little bits of circular DNA called plasmids.
Secondly, prokaryotes also have a cell wall, outside of the membrane.
And yeah, all cells are microscopic but there’s a big difference between eukaryotic cells and prokaryotic cells.
So eukaryotic cells, plant cells and animal cells, can be anything from 10 to 100x larger than prokaryotic cells, found in single celled organisms like bacteria.
I’m Dr Alex Lathbridge and this is Bitesize Biology. To hear more, search Bitesize Biology on BBC Sounds.
Listen on BBC Sounds
Question
What four things are found in nearly all cells?
Nearly all cells possess a membrane, a cytoplasm, DNA and ribosomes.
Episode 2 - Stem Cells
This episode breaks down the key facts about stem cells: what they are, how they differ in plants and animals, and why they're really useful for scientists.
Hello, I’m Dr Alex Lathbridge and this Bitesize Biology.
This is the second episode in a nine-part series on The Cell.
In this episode, we’re going to learn about stem cells: how they are different in plants versus animals and why they are really useful for scientists.
But before we get into that we need to back up a little.
All living things are made of cells. Remember, they’re the building blocks of life. (If that doesn't sound familiar, go back and listen to Episode 1 again.)
Plants and animals (like us humans) are made up of combinations of thousands of different types of cells, all working together to make the organism thrive. Remember the word organism. It just means an individual living thing like a plant or animal or human.
You're going to hear it a lot and examiners like it – so write it down:
Organism. I’ll give you an example: each human is an organism made up from about 200 different types of cell, these are things like blood cells, muscle cells and nerve cells.
Humans began in the womb as a fertilised egg cell or ovum called a zygote. One single cell.
And that means that those hundreds of different cell types all come from the division of that original cell.
So how do we get from one, single, fertilised egg cell to the complex being that you are?
It's all to do with stem cells. This process is important to remember. Grab a pen and make some notes.
We start with the zygote, the fertilised cell or ovum. This is a stem cell. An undifferentiated cell.
As that cell divides, it becomes what’s known as an embryo, that’s a small clump of stem cells.
Key here is that these embryonic stem cells have the potential to become anything that the body needs.
As you develop and grow in the womb, they receive signals that tell them to switch on certain genes, or differentiate, to become specialised for a specific role.
And that makes sense, because the cells in your eyes need to be very different from the ones in the skin.
This process, where cells change in different ways to have a specific job is called differentiation.
You need to remember that cells differentiate to become specialised.
Stem cells haven't been through this process yet, so they are undifferentiated.
Just imagine that all of your cells are being laid out on a family tree.
The trunk of the tree would be the fertilised egg and the leaves would be all of the 200 or more types of differentiated cells.
And in between the leaves and the tree trunk are the branches, our stem cells.
(Why aren’t they called branch cells? Yes, that would have made things far easier but some of the first work came from German scientists and they probably thought the word Stammzelle was cool).
You start with one undifferentiated stem cell. That’s a fertilised egg cell, a zygote.
This divides into an embryo, a clump of stem cells. So you’ve gone from a zygote into a clump of stem cells.
As the embryo divides, grows and develops in the womb, the stem cells receive signals that cause them to differentiate, to become specialised for a specific role.
This is complicated stuff so don’t worry if you don’t get it first time round. Listen back a few times, and remember you can always pause if you need to make notes, or ask a friend a question.
So those are embryonic stem cells.
Adults also have stem cells, called adult stem cells. We need them because we still need new cells to help us heal from injuries as well as replace our cells when they wear out.
Adult stem cells are left over from the embryonic stem cells that didn’t differentiate and they can only specialise into a few different cell types, unlike embryonic stem cells that can do all of them.
These are only found inside certain places: like the brain, bone marrow, blood, muscle, eyes, skin, liver and heart.
Stem cells are pretty useful inside us humans, but they are also useful outside the body.
Scientists can take stem cells and encourage them to grow into different types of cell, which we can then put back into humans, transplanting them into the bodies of people who need them to replace their own cells because they have become damaged.
Scientists are finding out more and more each day about the ways they might use stem cells to treat all kinds of diseases.
In terms of treating disease, adult stem cells are useful, to a certain extent, because although they can differentiate, they can only specialise into a few cell types, so they have got fewer options for what they can be.
For example, stem cells found in the bone marrow can be used to make new blood cells.
This means that a person could donate their bone marrow to someone who has a blood cancer like leukaemia. This bone marrow would be transplanted into the donor and, if successful, they would then make new, healthy blood cells to replace the damaged ones.
Now this science is relatively new and there are some issues.
Not everyone might want to donate their bone marrow and even if they do, not all donations are successful.
We could use embryonic stem cells, but many people question whether it is right to use human embryos for scientific research.
These are examples of ethical arguments which are constantly being debated.
Despite this, we’ve made brilliant strides. Researchers are even trying to work out how we might be able to turn regular cells back into stem cells, opening up potential ways to develop artificial organs and heal major injuries in the future.
Finally, you also need to know about stem cells in plants, because they work slightly differently from the ones in animals.
Stem Cells in plants are only made in special parts of the plant: the tips of roots and shoots called meristems (which makes it easy to remember).
The cells made in the meristem can differentiate to become any of the kinds of cells that the plant will need over its lifetime. This is why you can take cuttings of a plant and use that to grow a whole new plant.
You couldn’t grow a human from a chopped off finger.
I’m Dr Alex Lathbridge and this Bitesize Biology.
To hear more, search Bitesize Biology on BBC Sounds.
Listen on BBC Sounds
Question
How are embryonic and adult stem cells different?
Embryonic stem cells are present in zygotes and embryos. They can change into any cell type in the human body. Adult stem cells are only found in specific places (like bone marrow) and can only turn into a limited number of cell types.
Episode 3 - Specialised cells
This episode explores the key facts about specialised animal and plant cells, including: nerve cells, muscle cells, and sperm cells in animals, and root hair cells, xylem cells and phloem cells in plants.
Hello, I’m Dr Alex Lathbridge and this is Bitesize Biology.
This is the third episode in a nine-part series on The Cell.
So far in this series on The Cell we’ve talked about what’s inside the cell, and stem cells (undifferentiated cells.)
Let's flip it on its head, in this episode we’re going to talk about specialised cells, the differentiated cells, the cells that do a specific job.
There are six key cells you are going to need to know, so that’s what we are going to focus on today.
In animal cells, those are nerve cells, muscle cells, and sperm cells.
In plants, it’s root hair cells, xylem cells and phloem cells.
When you’re given questions about specific cells, there are two things you need to remember.
I call them the two Fs: function and form.
Basically, what does it need to do? Function. And what has it got to do it? Form.
So today, we’re going to be applying that to just six cells: nerve cells, muscle cells, sperm cells, root hair cells, xylem cells and phloem cells.
First, nerve cells.
Like your phone or laptop, your body relies on electricity, and it’s the nerve cells that carry electrical impulses, to transmit signals from your sense organs to your spinal cord and brain, and back to your muscles or glands.
Signals are passed from one long nerve cell to the next. Think of it like a baton in a relay race passing from one person to the next.
They send and receive electric signals as messages (or nerve impulses) these go from one nerve cell to the next, and eventually, to muscles and glands.
If you didn’t have a functioning nervous system, you wouldn’t be able to think, feel, or move, so they’re very important
How do they work? Well, nerve cells are long. This is so they can be in contact with the brain and spinal cord and the rest of the body.
They have a main cell body containing ribosomes and the nucleus, and if you can’t remember what ribosomes do, go back to Episode 1, have a listen and I’ll meet you back here.
Attached to the main cell body are dendrites and a long cable called an axon, covered in a fatty, myelin sheath to speed up the messages.
Number two. Muscle cells.
These are how we move when our muscles contract and relax to pull on our bones.
You know how you need to make sure you eat enough protein to help develop your muscles?
That’s because muscle cells are made up of proteins that slide over each other and contract to move.
We have three different types of muscle cell: skeletal muscle, smooth muscle and heart muscle.
These cells are powerful because they have a lot of mitochondria to release the energy to help you lift things (skeletal muscles), do a poop (smooth muscles), and keep your heart beating (heart muscles).
Third up, and the last one for animal cells, is sperm.
Sperm cells are genuinely the perfect example of understanding form and function.
So, what is the job of a sperm cell? Swimming to an egg cell, or ovum, and fertilising it.
And the sperm cell looks like a tadpole, with a head, a midsection and a tail.
In the head of the cell, there’s a cytoplasm and a nucleus, but also something really cool.
At the tip at the head of the sperm cell, there’s an acrosome (you don’t need to remember the name) but, it acts like the tip of a heat seeking missile, because inside it’s got enzymes that break down the jelly wall of the egg cell, so that the sperm can get inside.
Now we’re going to get into it, in a bit more detail in a later episode, but for now you need to know that the nucleus in the sperm only has half the number of chromosomes as a regular cell. This is because the egg cell is going to supply the other half.
The middle of the sperm is full of mitochondria, this releases the energy that powers the tail to swim towards the egg.
Because scientists like long, complicated words, the long tail of the sperm cell is called the flagellum, and it only allows the sperm cell to move forwards like a spinning missile towards the egg.
Alright, we’re halfway there. Those three, nerve, muscle and sperm cells, are specialised cells in animals.
Now we’re going to look at plants.
Plants don’t move to get food like we do so they don’t need muscle cells.
Plants need different types of cells and they’re all interlinked.
Cell number four is a root hair cell.
Root hair cells are how plants take the things they need from the soil, like water and nutrients.
They need to have a large surface area so they get in between the soil particles and absorb as much as possible (like a really large towel.)
The long part of the cell is what penetrates into the soil, sort of like a tent peg. This cell has a really thin cell wall, that allows for nutrients and water to pass in easily.
And like some of the other cells we talked about, they also have lots of mitochondria, for the energy they need.
But where does all that water go? Well, this is where cell number five, the xylem cell comes in.
Xylem cells need to contain water and transport it around the plant.
They have strong and study walls and stack on top of one another. Together they work kind of like a drinking straw. One long column of dead cells with their ends eroded away, so they can transport water, it’d be a waste of energy if these cells were alive and needed feeding.
Our sixth and final cell is the phloem.
Now if xylem is the water tube, phloem is the food tube. It transports sugars and water from the parts of the plant that generate food to the rest of the plant.
Because this a huge task, it’s joined by companion cells that have lots of mitochondria because it takes lots of energy to move this sugar.
I’m Dr Alex Lathbridge and this is Bitesize Biology.
All episodes are available on BBC Sounds.
Listen on BBC Sounds
Question
Why might a sperm cell have more mitochondria than an (ovum) egg cell?
Sperm cells need lots of energy to swim to the ovum (egg) to fertilise it. Energy is released during respiration which happens in mitochondria. So cells that need more energy have more mitochondria.
Episode 4 - DNA and the Genome
Dr Alex Lathbridge breaks down the key facts about DNA: what it is, how it's made and how it works. Dr Alex talks about how chromosomes, genes and nucleotide bases all work together, and what the genome is.
Hello. I’m Dr Alex Lathbridge and this is Bitesize Biology.
This is episode four of a nine-part series on The Cell.
In this episode we’re going to talk about a really important part of the cell, a really important part of your body, a really important part of you: DNA.
In the first episode about cell structure, I said, ‘almost all cells contain DNA.’
DNA is genetic material. Without DNA, there would be no life on earth, as we know it.
DNA is basically the instruction manual for building and maintaining a living organism.
So today, we’re going go into a bit more detail about what DNA is, how it’s made and how it works.
You might hear references to other things that we’re going to cover later in the series, like proteins.
So don’t worry if you leave today only knowing DNA.
It’s going to be useful for you to have the structure of the cell clear in your mind, so if you need a refresher, go back and have another listen to Episode 1.
Otherwise, grab a pen, let’s get into it.
DNA (or deoxyribonucleic acid) is a type of molecule known as a polymer.
This means it’s a large, complex molecule, made up of different, smaller chemicals called monomers that have been added together.
Top tip: the word “poly” means many and “mono” means one. Like a monorail has one track, a polygon has many different sides, monosyllabic is a single syllable and polymath is a person who knows many things, like you when you’ve listened to this podcast.
When it comes to monomers, you can think of them like beads on a string. You can add as many beads as you like, in whatever combination to make polymers.
And that makes them capable of holding information. And in the case of DNA, that information is the genetic code, the instructions to build a living organism, and more specifically, how to make proteins that help the cell do what it needs to do (but more on them later in the series).
DNA has four different 'beads' that you can choose from, these are monomers known as nucleotide bases, or just bases for short.
There are four different bases called: adenine, thymine, cytosine and guanine (or A,T,C,G.)
There’s a specific pattern: A matches with T and G matches with C.
It has to be this way because that gives DNA its structure, which you might have seen without realising, those tight twisted ladder shapes, known as the DNA double helix.
The bases are the rungs of the ladder. This is why when scientists talk about DNA, they refer to them as base pairs.
Weak hydrogen bonds form between A and T, and separately between G and C.
And the rope, in the DNA rope ladder, is made from alternating sugar and phosphate molecules.
DNA contains many important small sections called genes.
A gene is a small section of DNA that contains the code for a specific sequence of amino acids.
These are the building blocks to make proteins that the cell needs (but don’t worry – more on that later).
DNA is a polymer, made of 4 monomers called nucleotide bases: A, T G, and C.
DNA is incredibly long. Think about 3 billion base pairs per cell.
If you stretched the DNA in one cell all the way out, it would be about 2m long and all the DNA in all your cells put together would be about twice the diameter of the Solar System.
So you’re probably wondering how something that big could fit inside the cell, right?
Well, inside every cell is the nucleus, which basically like a folder for our DNA.
But the DNA is super tightly coiled, imagine your hair being twisted around itself until it’s barely noticeable. Same thing here, they’re known as chromosomes, your DNA tightly coiled around proteins and slotted inside the nucleus.
Most of your cells (there are some exceptions – but they are waiting for you in episode 6, so don’t worry about that right now) contain 46 chromosomes in 23 pairs.
Remember genes are heritable, you get one of each from each of your parents.
So, each gene contains a series of instructions for making a specific protein.
The DNA is arranged into chromosomes in 23 pairs, that’s 46 chromosomes in each cell.
Your entire genetic code, the complete set of instructions for how you grow, develop and function is called your genome.
I know it might sound difficult but here’s a good way of thinking about it (we’ll go smallest to biggest, and I’m hungry, so its food related.)
Bases are the letters.
DNA are like the words on a page.
Genes are the recipes.
Chromosomes are the cookbooks (of which you have 23 pairs – so 46 books in total.)
And the genome is the cuisine, like Ghanaian cuisine contains all the recipes that make up Ghanaian food.
There is something like 30 trillion cells in your body.
And nearly every cell contains the instructions for your entire genome.
Scientists have only recently worked out what this set of instructions is, this is the Human Genome Project, and it’s really exciting, cutting edge science, to help us find out how we evolved, how to treat diseases, and where we came from and where we are going.
I’m Dr Alex Lathbridge and this is Bitesize Biology. To listen to the rest of this series, and the other Bitesize podcasts, search Bitesize on BBC Sounds.
Listen on BBC Sounds
Question
Place these genetic structures in order of size starting with the largest: DNA base pair, chromosome, gene, genome.
The correct order is genome (one copy of all an organism's DNA), chromosome, gene (a section of DNA which codes for a protein) and finally DNA base pair.
Episode 5 - Protein Synthesis
This episode looks at how proteins are made during the processes of transcription and translation, using messenger RNA.
Hello, I’m Dr Alex Lathbridge and this is Bitesize Biology.
This is the fifth episode of a nine-part series on the cell. Today, we’re going to find out about protein synthesis.
You’re going to need to know some information from previous episodes on DNA and the genome, so go back and listen to that a few times to let in sink in. I recommend starting with cell structure and working your way from there.
I should probably start off explaining what protein synthesis means.
Honestly, it’s just a fancy way of saying: this is how your cells make proteins.
And it is really important that your cells make proteins.
Because I’m not joking when I say that proteins make everything in your body happen.
Imagine proteins as being like little machines inside your body, that are built for specific tasks.
Off the top of my head: some are used to break molecules down or stick them together (like certain enzymes, enzymes are proteins), some transport molecules around the body (like haemoglobin that carries oxygen in your red blood cells, haemoglobin is a protein), and if you squeeze your hands super tight right now, that sense of pressure that you’re feeling is thanks to sensory molecules in your skin, they’re proteins.
Even you, being able to listen to my voice right now, is thanks to a whole host of proteins in cells in your ears.
"But Alex, what’s the difference between protein in food (like chicken or tofu) and the proteins in my body?”
Great question with an easy answer: absolutely nothing.
The proteins that your cells need and the proteins in food are different, but they're made from the same building blocks: molecules known as amino acids.
Your body can break food down and add those amino acids that you consume, to make the proteins that it needs.
And because things are always happening in your body, your cells always need new proteins to replace the old, and that’s where protein synthesis, or making proteins, comes in.
In the last episode, we talked in a bit more detail about what’s inside the nucleus of the cell.
The nucleus is where the genetic material is kept. This genetic material, or code, is made up of a molecule called DNA.
DNA, or Deoxyribonucleic acid.
I said it might help to think about genetic material like a cookbook.
The DNA are like words, each gene is a recipe, and the chromosome is a cookbook.
A gene is a section of DNA with the recipe, or instructions, for making one protein (synthesising one protein.)
So, we know that making proteins, or protein synthesis, is important and we know that the instructions for making the protein are written in DNA, and this DNA is inside the nucleus.
The DNA is in the nucleus. But the protein itself is made somewhere else in the cell: the ribosomes.
If we think about the cookbook again, it’s a bit like having the cookbook in the bedroom when you need it in the kitchen. Easy, you might think, just take the cookbook from the bedroom to the kitchen.
But we have a problem. The cookbook contains so many recipes, that it’s just way too heavy, it’s too bulky, it’s too big to fit through the door, it can't leave the bedroom.
The solution? Just make a copy of the recipe you need and take that to the kitchen.
So you don’t need all of that genetic material, to make one protein you just need the code found in one gene.
Which is exactly what protein synthesis is: copying a single set of instructions and sending them to be made, or, in more scientific terminology that makes it sound way more complicated than it actually is, it’s known as transcription and translation.
Because you shouldn’t be going on about cookbooks in your exam, this is the process you need to know.
It’s in two stages so here’s where you grab a pen.
Stage one. Transcription.
Inside the nucleus, the section of DNA where the gene is gets unzipped (literally, two strands of DNA get split apart for a little bit) and a copy of the DNA sequence is made.
This copy is a molecule called messenger RNA (or mRNA) and it’s the transcript that will be used to make the protein.
Transcription is just copying the instructions in the gene into a different format that the ribosomes can easily understand. The ribosomes need to understand it, because they are where the protein gets synthesised.
Stage Two. Translation
The copied code, the instructions, the mRNA, leaves the nucleus, and travels into the cytoplasm where it joins with the ribosomes, those little protein-making robots, they read the copied code and they begin to create proteins, placing one amino acid at a time in a specific order, like beads on a string that then fold up into interesting shapes.
And that’s it: stage 1, transcription, inside the nucleus, making a small copy of the gene into mRNA.
And stage 2, translation, the copied code, the mRNA leaves the nucleus and goes to the ribosome where a protein is made. And after all this, the mRNA is broken down. It’s not needed anymore, mRNA is very, very short lived.
I know you’re probably wondering why I’m so hyped about this stuff, but I just think it’s really cool that millions and millions of years of evolution have given our cells the ability to make everything they need out of basic building blocks.
I’m Dr Alex Lathbridge and this is Bitesize Biology. The rest of this series on the cell, and the other topics that we cover, are available on BBC Sounds.
Listen on BBC Sounds
Question
What are proteins made from?
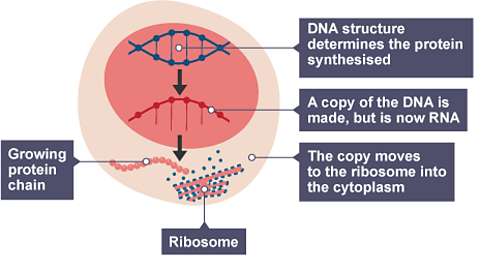
Proteins are chains of amino acids folded into the correct shape.
Episode 6 - Mitosis and meiosis
This episode covers mitosis, which involves interphase, prophase, metaphase, anaphase, telophase and cytokinesis.
Hello, I’m Dr Alex Lathbridge and this is Bitesize Biology.
This is episode six in our nine-part series on the cell.
Today, we’re going to find out about cell division, basically how cells divide themselves to grow and repair damage and keep the same genetic material along the way.
We’ll talk through the stages of the cell cycle and how some cells doing things a little bit different makes us all unique.
This is all to do with DNA. So, if you need a little reminder about DNA and the genome, you can jump back and have a listen to episode 4 that before we get into today.
Every cell in your body has been made by some other cell. And it’s important that the new cells have the same genetic material as the old ones.
Because that’s the thing, for you to get taller as you grow up or for your skin to heal itself if you get a cut, new cells have to be perfect copies of the old ones.
This is down to a process called mitosis.
In the most basic terms, mitosis in plants and animals, including humans, can be summed up as:
A parent cell grows bigger, copies its DNA, and splits in two to make two new daughter cells.
Is there a little bit more to it than that? Yeah, there are like 6 minutes left on this thing.
Now mitosis happens as part of the Cell Cycle.
You need to remember the stages, so grab a pen and write this down.
The cell cycle can be broken down into six key stages:
Interphase, Prophase, Metaphase, Anaphase, Telophase, and Cytokinesis.
Interphase is always at the start, Cytokinesis is always at the end, and the four stages in the middle are what makes up mitosis.
So, let’s go through this six-part cell cycle.
Interphase. The DNA copies itself and the cell enlarges.
Prophase. The cell prepares itself by condensing DNA into chromosomes and breaks down the nucleus.
Metaphase. The chromosomes line up across the middle of the cell.
Anaphase. The chromosomes are pulled to the opposite ends of the cell.
Telophase. Two new nuclear membranes form around the chromosomes at each end of the cell.
Cytokinesis. The cell divides down the middle to become two new cells. We call these daughter cells.
The first part of the cell cycle is a stage known as Interphase.
Most of the cells in your body right now are in Interphase. You can think of interphase as the in between phase. It’s a time when cells go through their normal processes. It’s the part of the cell cycle where the cells aren’t dividing but they are getting ready for it.
The cell grows in size and copies everything. That means DNA inside the original cell’s nucleus replicate and are checked for errors.
Next up is the first stage of mitosis: Prophase.
The way I remember this is that prophase is your prep phase.
DNA strands become more visible when they condense, forming really compact, tightly twisted structures known as chromosomes – containing all of the cell’s genetic data (or genome). As well as that, the membrane around the nucleus breaks down.
Next up is Metaphase. I call it the “middle phase” of mitosis, because all of chromosomes line up along the middle of the cell.
Next up is Anaphase. Chromosome pairs get pulled apart from the middle to opposite ends of the cell and stay there. One copy of each pair is at the end of the cell. So, each side of this large dividing cell has a regular amount of chromosomes for one cell.
Next, we have Telophase. This is where new membranes form around the chromosomes at each end of the cell. One cell with two new nuclei.
So that’s mitosis over, but how does one large cell become two daughter cells?
This is Cytokinesis. This is where the cell membrane pinches in and eventually divides into two daughter cells.
And because it’s a cycle, it’s straight back into interphase to happen all over again.
If mitosis is all about making identical genetic copies, what happens when you need some variation in cells?
You get half of your DNA from each of your parents. Half from egg cells, or ova, and half from sperm cells. So why don’t people with the same biological parents look exactly alike?
Because, if you have siblings, one of you might have your dad’s chin and the other might have your mum’s nose.
This is because, genetically speaking, no two egg cells from a person are likely to be the same, just as no two sperm cells from someone are likely to be the same. But how?
It’s the next process you are going to need to remember: meiosis.
Generally speaking, every cell in a human body (apart from red blood cells, but don’t worry about them for now) contains 46 chromosomes and these chromosomes are in 23 pairs.
Cells with 23 pairs of chromosomes are known as diploid (you can think double, paired.)
But reproductive cells, called gametes, like eggs and sperm cells are different. They’ve only got one set of chromosomes and they aren’t paired. So, 23 chromosomes. They’re known as haploid (or half, unpaired.)
Egg cells and sperm cells merge in fertilisation. They combine their 23 chromosomes, ending up with 46 chromosomes in total.
Like in mitosis, the way most cells copy themselves for growth and repair, meiosis starts with Interphase, where the cell doubles the amount of DNA within.
In Prophase, it gets different. Because in meiosis, some sections of DNA are randomly exchanged between the different chromosome pairs, after they’ve condensed. This creates a unique set of chromosomes that has never existed before. Think of each chromosome pair that’s doing this as being like two decks of cards being randomly shuffled together.
Then it’s pretty much like mitosis. Chromosome pairs line up, they get pulled apart, form new nuclei and divide into two.
Like mitosis, this creates two new cells. But they’re still diploid cells, they’ve got 46 chromosomes in 23 pairs.
So, this means that each new cell has to do another cell division. Because, gametes are sex cells, they need to have 23 chromosomes only.
But - and this is key – without interphase – so no DNA copying itself and no shuffling over DNA again.
So after the end of this second round of division, we’re at the end of meiosis and we have created four, haploid sex cells from one diploid parent cell.
Ok – let’s go through that again
Step 1. The chromosomes make copies of themselves
Step 2. Chromosome pairs line up
Step 3. Sections of DNA get swapped like two decks of cards being shuffled together
Step 4. First cell division: chromosome pairs separate
Step 5. Second cell division: DNA is now half that of a normal cell.
So now you’ve got four cells, each with a single set of chromosomes. These form gametes, your sperm or eggs.
I’m Dr Alex Lathbridge and this is Biology Bitesize. All episodes available on BBC Sounds
Listen on BBC Sounds
Question
Describe the cells formed at the end of mitosis and meiosis.
Mitosis produces two genetically identical daughter cells. These are body cells so are diploid. Meiosis produces four genetically different daughter cells. These are gametes (sperm or ova) so are haploid.
Episode 7 - Diffusion, osmosis and Active transport
In this episode, Dr Alex Lathbridge breaks down the key facts about how things move in and out of cells.
Hello, I’m Dr Alex Lathbridge and this is Bitesize Biology.
This is the seventh episode of a nine-part series on the cell.
This episode is all about how things move in and out of cells through the cell membrane. There are three main ways that this happens and we’re going to cover them all today: diffusion, osmosis and active transport.
Things need to move in and out of the cell to keep the organism alive. It helps keep the balance of molecules needed for survival, such as oxygen and water.
You’re going to need to be able to identify which kind of movement is happening in a cell and to help you work that out there are two questions you should be asking yourself: what is the direction of travel and which molecules are doing it?
Process number one is called diffusion.
Imagine you’re at a party. A gathering of people in a generally fun space.
You’re in the corner of the room because it’s a bit too crowded, so you want to move across the room to where there are fewer people.
This is basically diffusion, it’s the gradual movement of things from places where there are lots of them, to places where there are fewer of them, until it all evens out.
For you to be able to party, of course you need to pass your exams so, in biological terms, the people at this party are called particles, things like molecules and ions.
And the room that you’re in is a liquid or gas.
The movement is happening all the time, and while one particle can go to an area where there are lots of other particles if it really wants to, over time they naturally move from crowded areas to less busy areas.
This is basically what’s known as a concentration gradient, an environment where you have a high concentration of particles in one area, but a lower concentration in another.
In basic terms, its going from a place where there’s lots of a particle, to a place where there’s not so many.
This movement, this process of diffusion is important, because it’s how glucose and oxygen move into the cells, and the cells need glucose and oxygen because they react to release energy.
And once they’ve released that energy, there is left over: carbon dioxide. And the cell needs diffusion to make that leave the cell. (That energy stuff is waiting for you in episode 9).
This is important so I’m going to recap that.
Grab a pen so you can write this down.
Diffusion is how particles, molecules and ions, move from higher concentration to lower concentration so they can spread out evenly through a liquid or gas.
This is for carbon dioxide, water, urea, food and waste.
Diffusion is how glucose and oxygen move into cells so they can release energy.
The process of releasing energy also makes carbon dioxide, which leaves the cell using diffusion.
Diffusion can't occur in solids because the particles are not free to flow like liquids and gases.
Alright, so that’s diffusion done.
The second type of movement you need to know is osmosis
But first, let’s talk about orange squash for a bit. It is relevant, I promise.
If you add lots of water to your orange squash, it becomes lighter in colour. You’re diluting it.
A dilute solution contains a lot of water. But if you only add a tiny amount of water, then it’s concentrated.
This you can think of as a solution, which is water plus other stuff.
Why is squash relevant to osmosis?
Well, osmosis is all about water molecules in solutions. It’s a special type of diffusion just for water. Imagine it as diffusion through a sieve.
So, in animals your cells have dilute solutions of ions, sugars and amino acids.
The cells need these things and when they are mixed with water it means that they can enter and leave the cell when they are needed.
The same principle as diffusion applies: ions, sugars and amino acids move from areas where there are lots of them to areas where there are fewer, making it all even out.
This happens through something called a selectively or partially permeable membrane.
This is a membrane that allows some things to pass through but not others, small things but not big things, like a sieve.
Remember that plants have a cell wall keeping them upright? (If you don’t, have a listen again to episode 1 to refresh your memory of the basics.)
Well, the cell wall is fully permeable, which means that it lets in and out all molecules that the cell needs or doesn’t need any more.
But when it comes to animal cells, including human cells, we don’t have cell walls, which means that water makes our cells change size and shape and they could burst if they take in too much water by osmosis.
So it has to be strictly controlled otherwise they can shrink or worse, they can burst and become permanently damaged.
To recap: in osmosis, water moves from high to low concentration through a semi-permeable membrane.
So, from a dilute solution with lots of water in it, to a concentrated solution with less water in it.
Our third and final process is known as active transport.
It’s called active because it needs energy to make it happen.
The other types of movement, diffusion and osmosis, are called passive transport, which means they happen without the cell needing any energy to do it.
Active transport happens when molecules need to move from a place of lower concentration to a place of higher concentration.
Think back to plants. They’ve got root hair cells that take minerals up from the soil into the plant.
So, at a certain point, there are going to be more minerals inside the plant rather than outside.
But active transport allows the plant to keep taking those minerals in.
But what about in animals?
The carbohydrates in your food are digested into glucose. The glucose then needs to be moved from the small intestine to the blood.
All the glucose needs to go, but sometimes you might not have that much left, meaning there is more glucose in the blood, than remains in the small intestine.
So here the cell uses energy, active transport, to help get that glucose out over the cell membrane and into the blood.
I’m Dr Alex Lathbridge and this is Bitesize Biology. All episodes available on BBC Sounds.
Listen on BBC Sounds
Question
How is active transport different from diffusion and osmosis?
Active transport is the only process which moves particles from low to higher concentration and so requires energy.
Episode 8 - Photosynthesis
Plants make their own food using photosynthesis. This episode explores how plants and algae make their own food by taking energy from sunlight to make glucose. Dr Alex also takes a look at the photosynthesis equation and the factors that affect the rate of photosynthesis.
Hello, I’m Dr Alex Lathbridge and this is Bitesize Biology.
This is the eighth episode in a nine-part series on the cell.
In this episode we’re going to find out about photosynthesis, one of the most important processes to happen inside plants and one that changed life on Earth.
A few billion years ago, some early microscopic organisms that lived in water said:
“You know what, it would be really good if there was some way that we could use that big, burning ball of fire up in the sky, to keep ourselves alive. It would make it kind of easy for us to evolve into plants, that could live on land, that could grow tall, and release oxygen into the atmosphere so maybe complex lifeforms could exist. Maybe they could eat us? Just saying, it’s literally right there.”
And evolution said: “Yeah, I can’t lie, that’s actually a very good idea. Wait a couple of billion years.”
I don’t think you’ll pass if you put that on the exam, so grab a pen and let’s get into the ins and outs of photosynthesis.
Photosynthesis is how plants and algae make their own food by taking energy from the sunlight and using it to make a sugar called glucose.
They then use some of this glucose to make the larger, more complicated molecules they need to grow.
You’ll need to remember the names of the things plants have to do this, so it’s a good idea to make some notes or draw your own diagram.
Photosynthesis mainly happens in leaves of the plants.
Cells in plants have things called chloroplasts. Chloroplasts contain a chemical called chlorophyll, which is what they use to absorb light from the sun.
Easy way to remember: chloroplasts are filled with chlorophyll.
Fun fact: chlorophyll is what gives plants their green colour and if you look at the chemical structure of chlorophyll, it looks like a flyswatter.
The main place that you will find chloroplasts are in two types of cells with weird names:
Palisades and spongy mesophyll cells.
As well as light from the sun, plants need two other things for photosynthesis:
They need carbon dioxide; they take this from the air through holes in the leaves called stomata.
And they need water, which they get from the soil and is transported through the plant by its plumbing system - the xylem. (And if the word xylem doesn’t ring any bells, go back and listen to Episode 3 for a bit more info.)
So, plants take light from the sun and use its energy to convert carbon dioxide and water into glucose, a sugar.
They also use this energy to make molecules such as cellulose, for cell walls, starch for storage, and amino acids to make proteins.
Humans can’t perform photosynthesis. We don’t have any chloroplasts or chlorophyll, so we aren’t able to convert light from the sun into chemical energy.
However, it’s still good news for us that plants photosynthesise because plants store some of the energy in the form of glucose inside the plant and when you eat that you get the energy. Which makes eating lettuce a lot more interesting.
There is an equation for photosynthesis that you are going to need to remember so write this down:
Carbon dioxide + water is converted into glucose and oxygen.
Or 6CO2 + 6H2O -> C6H12O6 + 6O2
Six carbon dioxide molecules plus six water molecules is converted into a glucose molecule, plus six oxygen molecules.
When I say converted, when you’re writing an equation you show a long right arrow.
This is an endothermic reaction, what that means is that it absorbs energy from the environment to make it happen. The easy way to remember this is that the sun gives off a lot of heat for the plant to use.
There are three factors that are important when it comes to the rate of photosynthesis:
The amount of carbon dioxide.
The heat of the environment
And the amount of light.
These three factors affect the rate of photosynthesis, which means that if any of these factors aren’t at the right level, it can slow down photosynthesis and then it becomes the limiting factor.
If there’s lots of light and a high concentration of carbon dioxide, but it’s still going slowly, chances are that temperature is to blame.
If the temperature is fine, and there’s lots of carbon dioxide, chances are there’s not enough light. So, the limiting factor can change.
I’m Dr Alex Lathbridge and this is Bitesize Biology. Subscribe and download the rest of the podcast episodes BBC Sounds.
Listen on BBC Sounds
Question
What is the equation for photosynthesis?
The correct equation is carbon dioxide + water → glucose + oxygen. Remember you can swap the order of carbon dioxide and water (but they must stay before the arrow) and glucose and oxygen (after the arrow).
Episode 9 - Respiration
Respiration is a chemical reaction which occurs in all living cells, releasing energy from glucose. Dr Alex Lathbridge breaks down the key facts about respiration: how living things break down glucose to release energy, carbon dioxide and water.
Hello, I’m Dr Alex Lathbridge and this is The Bitesize Biology Podcast.
This is the last episode in our nine-part series about the cell.
And well done us because we’ve gone through the structure of the cell, how cells specialise to do different jobs, how cells make proteins, and how cells control what goes in and out, and how DNA are the instructions for life.
But there’s one more cellular process that you need to know about: respiration.
First, we’re going to start with what respiration isn’t.
Respiration isn’t about cells breathing, I know it sounds like it, but in this case, it isn’t.
It is about how living things get their energy from food. Respiration is how the energy is transferred by breaking down the sugars, so your cells can use the energy stored in there. You’ll need to remember the two different types of respiration with similar names:
aerobic and anaerobic respiration – with oxygen and without oxygen.
So grab a pen and write this down:
Your cells need energy for all of the things they need to do to keep you alive, for movement, growth and repair.
Warm blooded creatures like you and me, need it to keep their blood warm.
When we eat carbohydrates, our body breaks it down into simple sugars. There are many sugars but the one you need to remember is glucose.
Your cells need the energy provided by glucose, but you can’t just pour syrup on your cells like they’re microscopic pancakes.
The cells need to turn it into something that the body can use.
All cells do cellular respiration because all cells need energy, and they’ve got two different ways of
doing it, both of which take place in the cytoplasm and mitochondria of the cell.
Cytoplasm is where the chemical reactions take place in the cell.
Mitochondria is where cells release the energy they need and where respiration takes place (you see why I called it the battery of the cell in previous episodes, yeah?)
Grab a pen, and let’s go.
The first one we’re going to chat about is aerobic respiration:
This is where the cell takes the glucose and it reacts with oxygen (which is where “aero” in the name comes from).
This releases the energy so the cells can use it.
There are also some waste products made, things that aren’t needed by the cell.
These are carbon dioxide and water, eventually expelled by the body.
There’s an equation, or formula, that you are going to need to remember (so please write this down):
glucose + oxygen → carbon dioxide + water and energy released
C6H12O6 + 6O2 → 6CO2 + 6H2O
Now if that sounds familiar, it's because when we go back to the photosynthesis episode, the photosynthesis equation is just this one backwards.
If you need some help remembering this, this might not work for you, but I remember it as 121 666.
Let me explain:
There are six oxygen molecules going in (6O2) and six carbon dioxide and six water molecules coming out on the other side (6CO2 and 6H2O).
Then just try to remember that glucose has six carbons, twelve hydrogens, and six oxygens (so if you divide by six – that’s one, a two and a one.)
glucose + oxygen → carbon dioxide + water and energy released
C6H12O6 + 6O2 → 6CO2 + 6H2O
Now remember when we say converted in equations you show that with a right-handed arrow, check out the Bitesize website and you can see that equation.
Don’t forget that plant cells respiration too. They can respire in the light and in the dark.
So most respiration needs oxygen, but not all, and this is where the second type comes in: anaerobic respiration.
Imagine that you’re going for a run, you're really, really pushing yourself. You’re working your muscles hard, and they need energy quickly. You are breathing in and out, but you can’t get the oxygen to your muscles fast enough, but you need to keep going and your muscles start to ache, they start to burn.
This is because your muscles are using anaerobic respiration.
Anaerobic respiration not ideal because it’s not very efficient at all.
It initially releases nineteen times less energy than aerobic respiration, but you can do it for short periods of time if you need to – if you need to finish that run.
And that ache in your muscles is because anaerobic respiration makes lactic acid.
This lactic acid builds up, and your body is not a fan of it, and this is why your muscles start to hurt.
Now your body has to get rid of that lactic acid, so it’s taken to the liver by your blood where it’s mixed with oxygen to turn into carbon dioxide and water, this releases the rest of the energy, or it's converted to glucose.
Both these methods of getting rid of lactic acid require oxygen, it's why you keep breathing really heavily after exercise.
While you don’t need oxygen to release energy in anaerobic respiration, you do need oxygen to get rid of the lactic acid it makes.
So in anaerobic respiration glucose is turned into lactic acid, which releases energy.
It's not just animals that do this, there is also a useful single celled organism called yeast that uses anaerobic respiration. We use yeast to make bread or alcoholic drinks like wine or beer.
Yeast reproduces rapidly, using up all the oxygen so it switches to anaerobic respiration which also makes ethanol and carbon dioxide. So, ethanol (alcohol) is a by-product of anaerobic respiration.
Let’s take a moment to compare them:
Aerobic respiration uses oxygen.
Anaerobic respiration can happen without it.
In aerobic respiration the oxidation of glucose is complete.
In anaerobic respiration it’s incomplete, this means that there’s extra stuff that it makes still containing energy.
So aerobic respiration makes carbon dioxide and water, and those things don’t have available energy, but anaerobic respiration makes lactic acid in humans and animals. In yeast it makes ethanol and carbon dioxide. These things still have some energy in them, so it’s not a very efficient process.
Aerobic respiration releases quite a lot of energy and anaerobic respiration only gives a small amount, but it happens very quickly, ideal if you are running away from something that wants to eat you.
One final thing you need to remember is that respiration is exothermic, this means that energy is released to the surroundings.
I’m Dr Alex Lathbridge and this is Bitesize Biology. You can listen to the rest of this series, and all the other topics that we’re covering on BBC Sounds.
Listen on BBC Sounds
How are aerobic and anaerobic respiration different?
Aerobic respiration occurs reacts glucose with oxygen and produces carbon dioxide and water. It is slower but releases more energy. Anaerobic respiration occurs without oxygen and produces lactic acid. It is faster but releases less energy.
Links to further learning for science GCSE
More on Podcasts
Find out more by working through a topic
- count2 of 7
- count3 of 7
- count4 of 7
- count5 of 7